Research Spotlights
Improving energy materials by understanding heat flow on the atomic scale By Georgia Barrington-Smith & Dr Rebecca Duncan With advancements in technology and increases in population leading to a looming energy crisis, it is vital to optimise energy use to meet our future needs. One of the major issues in …
By Georgia Barrington-Smith & Dr Rebecca Duncan Cancer continues to be one of the leading causes of death worldwide, claiming the lives of millions of people each year. One of the first-line treatments of cancer is chemotherapy: powerful drugs that attack cancer cells and prevent their spread. Historically, the main …
by Georgia Barrington-Smith, 31st October 2024 The Environmental History of the Great Barrier Reef, as told by a Giant Clam Shell Although archaeology has made waves on land, we know comparatively little about the history beneath our shores. A few centuries ago, the Earth experienced a Little Ice Age (LIA) …
by Georgia Barrington-Smith, 11th October 2024 How Pipelines Impact the Marine Food Web AINSE’s focus for October is Oceans Month, which we are kicking off by spotlighting Alexandra (Alex) Bastick: an Honours student from Charles Sturt University, AINSE Pathway Scholar, and emerging young voice in environmental research! With our oceans …
By Georgia Barrington-Smith
Anthropomorphic climate change is weakening the resilience of globally significant forests by altering their temperature and aridity. Tasmanian Wilderness World Heritage Area (TWWHA) is particularly at risk, with longer, more intense bushfire seasons threatening this sensitive ecosystem. Amid ongoing climatic stress and ecological decline, important questions are emerging around the ability of these ecosystems to persist in a changing landscape.
Various conservation efforts have been implemented to address growing concerns, including targeted burning to reduce fuel loads, the establishment of seed banks, and restoration planting. Unfortunately, these strategies have not proven effective in the long term, and implementing these measures is especially challenging in remote and environmentally-sensitive areas like the TWWHA.
To combat these challenges, scientists have investigated the specialised adaptations of existing fire-sensitive species, like the ancient Tasmanian Pencil Pine (Athrotaxis cupressoides), that are persisting in the face of an increasingly flammable environment. By looking to nature’s own solutions, scientists can gain critical insights to help develop more effective, long-term strategies for future bushfire management.
Pencil Pine serves as a proxy for forecasting future fire effects
Sarah Cooley, an AINSE PGRA scholar and University of Melbourne Ph.D. student, has used the endangered Tasmanian Pencil Pine as a target species to gain deeper insight into how climate-fire vegetation dynamics have influenced the response, resilience, and post-fire recovery of these ancestral tree species.
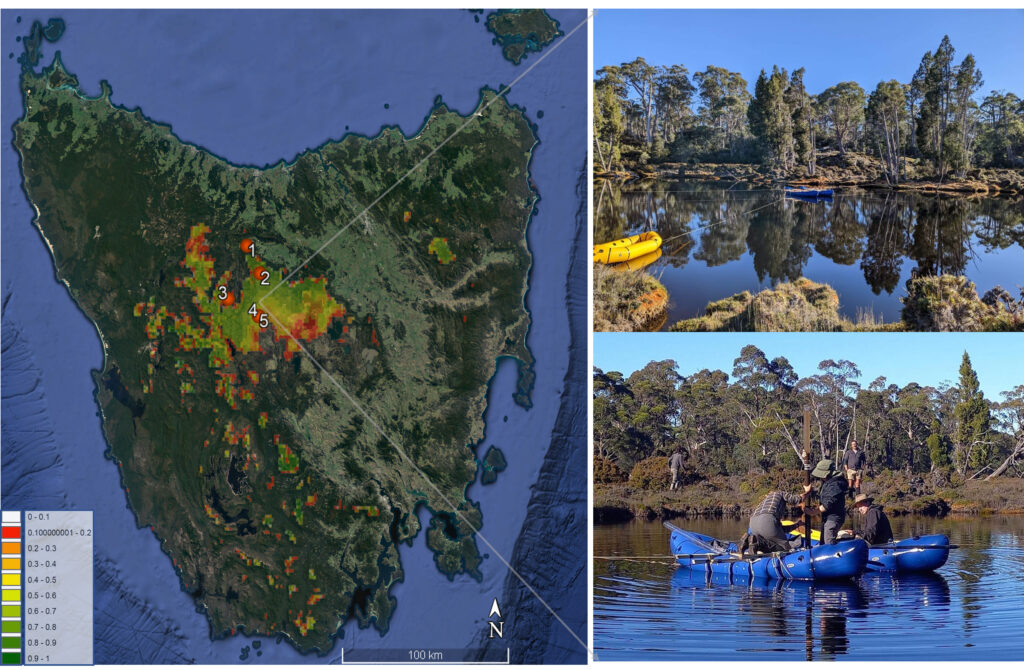
Sarah and her collaborators applied a multi-proxy approach by gathering multiple types of environmental and climate data from four sediment cores taken from lakes and bogs across Tasmania’s Central Plateau. The selected sites represent different levels of climate suitability for Pencil Pine growth today, based on temperature and rainfall patterns. This was determined using species distribution modelling (MaxEnt), which predicts where the Pencil Pine is most likely to thrive.
Their comprehensive environmental and climate record combined analyses of fossil pollen and charcoal (palaeoecology) with high-resolution stable isotope and trace element data from cave deposits (palaeoclimatology). A variety of advanced techniques were used to collect this data, including Itrax micro-X-ray fluorescence analysis at ANSTO’s Environmental Laboratory and radiocarbon dating at ANSTO’s Centre for Accelerator Science (CAS).
By analysing this detailed record, the team could illustrate how numerous interconnecting factors, including historical vegetation, fire, and catchment dynamics, all interact to influence the resilience and post-fire regeneration of these highly sensitive ecosystems.
Sarah’s contribution to fire management
Armed with this new information, researchers can adapt traditional fire management strategies to better preserve critical species in changing landscapes.
The detailed paleoenvironmental record developed by Sarah and her colleagues enables the development of advanced scientific models that can predict shifts in species distribution under a range of possible future climate scenarios. These models are essential for making well-informed decisions when managing and responding to fires in remote and vulnerable areas, such as the Tasmania Wilderness World Heritage Area.
With fire management strategies enhanced by these paleoclimate records, Australia’s ancient and significant plant species are better positioned for survival in the face of climate change.
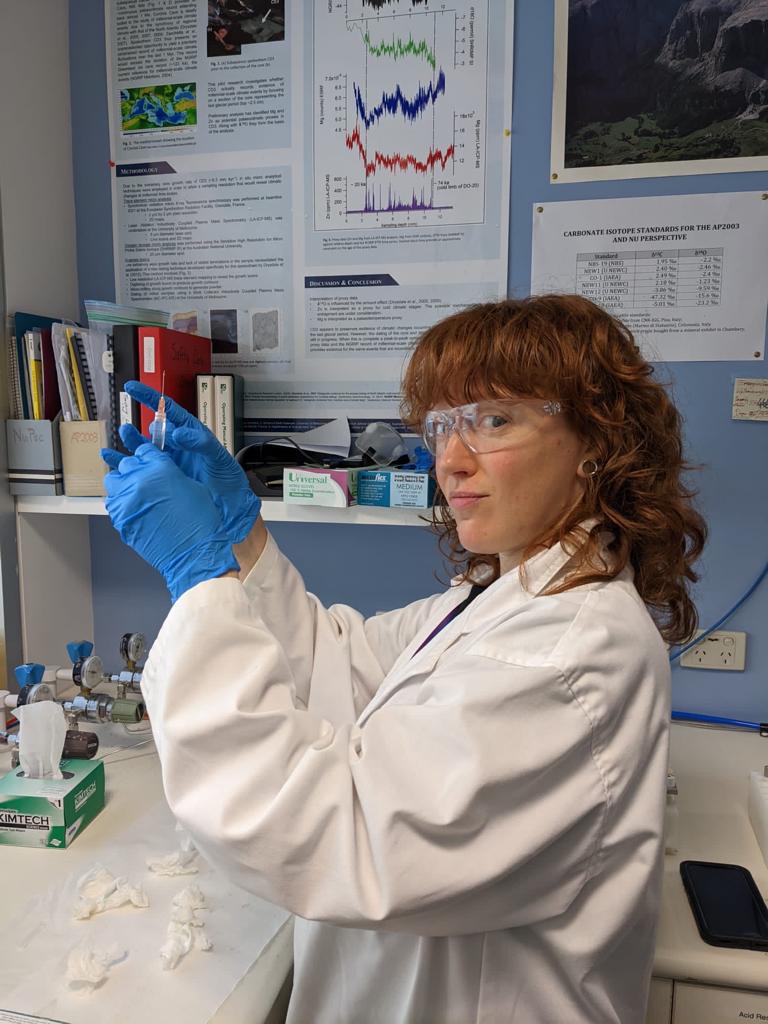
AINSE are proud to spotlight Sarah for her outstanding work!
If you, just like Sarah, are interested in researching the wonders of Australia’s wilderness, visit ainse.edu.au/scholarships to see how AINSE can support you.
Discover more research spotlights at ainse.edu.au/research-spotlight.
Stay tuned for our brand-new spotlight series launching next month as we get connected for Magnetic May! Kicking off the series is Marco Vás, whose research explores how structural and magnetic changes in materials can drive the next generation of electronics.
Follow ainse_ltd on Instagram, Facebook, Threads and LinkedIn to keep up to date with upcoming events and research spotlights.
By Georgia Barrington-Smith & Dr Rebecca Duncan
Anthropogenic-driven climate change has extended the duration of Australia’s annual fire seasons, wreaking havoc on agricultural crops, wildlife, and homes. The 2019-2020 bushfires, which scorched over seventeen million hectares and claimed the lives of over one billion animals, provide a stark example of this growing crisis.
Satellite observations can offer insights into past fire regimes, but such records are not extensive enough to capture the entire range of natural variability, or the feedback between climate, landcover, and human activities. Given that climate models forecast even more intense bushfire seasons in the future, it is critical that research continues to analyse historical data to track these changes. UNSW researcher Dr. Micheline Campbell, in collaboration with ANSTO, has examined the history of Australian bushfires etched in cave deposits (speleothems) to help strengthen our fire management strategies in the future.
The hidden history buried in speleothem caves
Speleothem caves contain stalagmites and stalactites – mineral formations created by groundwater that drips onto the cave floor. These mineral formations can be isotopically dated to determine past climatic conditions, including past bushfires.
Over thousands of years, as bushfires burned above, ash deposited over caves were embedded into speleothems by rainwater, preserving trace element layers of the ash. This process creates ‘paleofire signals’ that act as a chronological record of the environment above the cave, capturing yearly variations over millennia. Research by Dr. Micheline Campbell and her collaborators aims to reconstruct not just the timing, but also the severity of ancient fires by analysing these trace elements preserved in the speleothem archives.
Micheline’s historical hunt for fire information
Micheline and her colleagues began by collecting ash samples from recent bushfires in south-west and south-east Australia. At ANSTO’s Environment Research & Technology (ERT) laboratory, Micheline then performed uranium-series dating techniques on these samples to count their annual growth bands. By analysing this data, she showed that variations in ash metal concentrations were related to the burn severity of the associated bushfires.
Next, Micheline set out to determine whether the severity of burns indicated by ash data was similarly recorded in speleothems. To do this, she collected ‘mini cores’ from the actively-growing tips of stalagmites across different caves in Yanchep National Park, Western Australia – an area that has experienced a variety of bushfires to varying degrees.
Using the X-ray fluorescence microscopy beamline at ANSTO’s Australian Synchrotron, and laser ablation inductively-coupled plasma mass spectrometry at ANSTO’s ERT lab, the team created elemental maps of the samples. Their preliminary results revealed distinctive metal patterns derived from the ash following known fires.
By examining the ash composition and comparing it to stalagmites from areas with recorded fire events, Micheline and her collaborators discovered that the speleothems from Yanchep National Park in south-western Western Australia are likely to offer the sought-after high-resolution records of fire severity and frequency from our deep past.
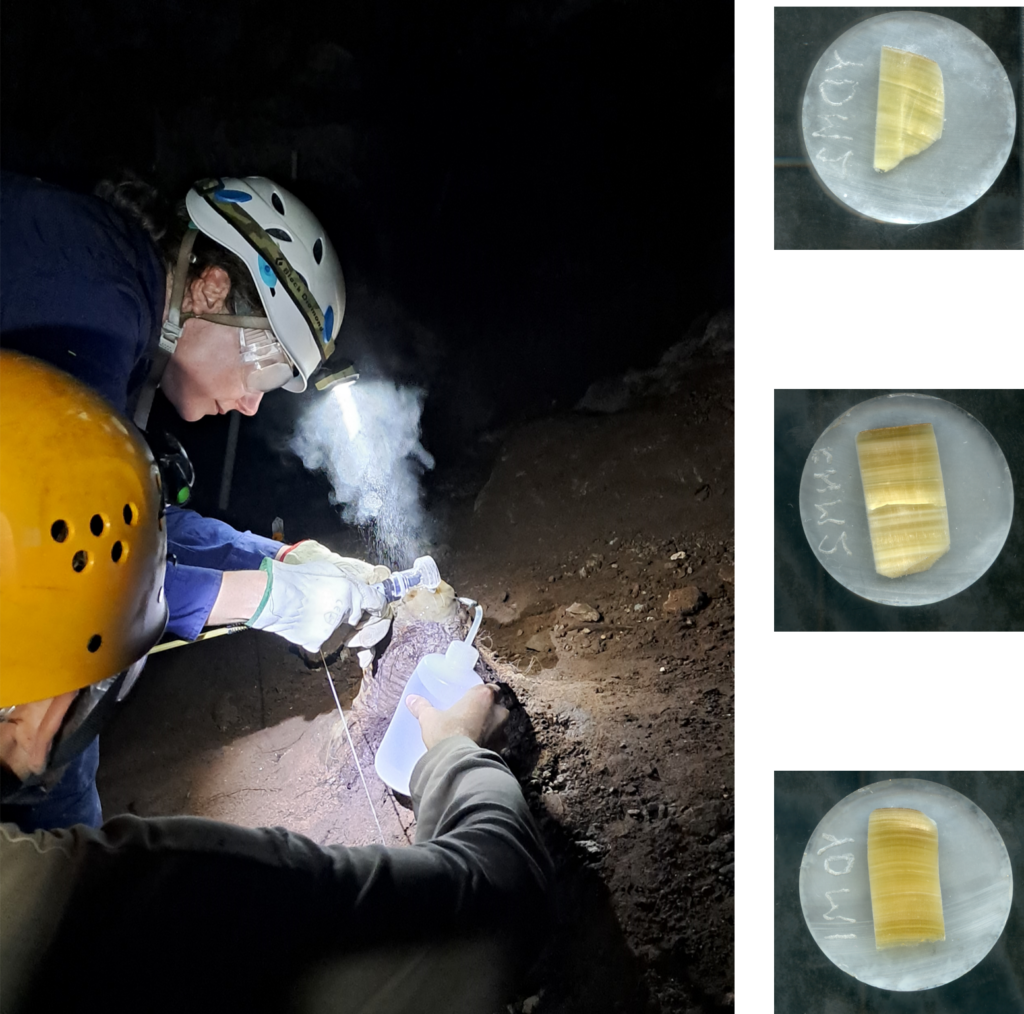
Historical insights guiding future decisions
Micheline’s research underscores the importance of analysing historical records to enhance our understanding of future environmental changes. The paleo-information archived in speleothem caves can provide significant insights for improving land management strategies, thereby enabling more informed decision-making in response to climate change.
This work is part of an Australian Research Council Discovery Project led by Prof. Andy Baker (UNSW), Dr. Pauline Treble (ANSTO), and Dr. Liza McDonough (ANSTO). It builds on previous studies conducted by ANSTO Environmental Scientist Dr. Liza McDonough, who identified the possibility of reconstructing fire events from stalagmites.
AINSE are proud to spotlight Micheline for her fantastic work!
If you, just like Micheline, are keen to discover the answers to some of Australia’s toughest environmental challenges, visit ainse.edu.au/scholarships to see how AINSE can support you.
To read more research spotlights visit ainse.edu.au/research-spotlights. Additionally, keep an eye out for the second article in our April Awareness issue, where we will showcase the work of Sarah Cooley, an AINSE PGRA scholar, who is using historical records of fire-sensitive Pencil Pine to study the bushfire resilience of Australia.
Follow ainse_ltd on Instagram, Facebook, Threads and LinkedIn to keep up to date with upcoming events and research spotlights.
Timeline
About AINSE
The Australian Institute of Nuclear Science and Engineering (AINSE Ltd.) is an integral organisation for enhancing Australia’s and New Zealand’s capabilities in nuclear science, engineering, and related research fields by facilitating world-class research and education.
AINSE offers a range of programs and services to its members, including generous domestic and international conference support, scholarships for honours & postgraduate students and Early Career Researchers, and intensive undergraduate education schools. These benefits aim to foster scientific advancement and promote an effective collaboration between AINSE members and ANSTO.
We respectfully acknowledge the Dharawal nation as the traditional custodians of the land on which AINSE is located.
Copyright © 2019 AINSE | Powered by Astra