By Georgia Barrington-Smith
In today’s digital era, the rapid proliferation of online services and cloud computing has driven an unprecedented global demand for data centres. These facilities support the storage and distribution of vast data volumes, ranging from AI models to high-demand streaming content.
Unfortunately, as our reliance on digital infrastructure and artificial intelligence accelerates, so too does its environmental footprint. Data centres, despite their benefits, consume vast amounts of energy to run. By 2040, the growing demand for energy by digital data storage alone could account for up to 14% of global carbon emissions.
Meeting this rising demand without overwhelming the planet’s resources requires bold innovation—starting at the very foundation of computing: the microscopic materials that drive our devices.
Beyond the limits of silicon
At the heart of modern electronics are semiconductors, tiny components that enable the function of everything from smartphones to supercomputers. Silicon-based semiconductors have been widely used for years, but their low energy efficiency and susceptibility to electrical leaks can limit their performance as demand continues to grow for smaller, faster, and more powerful devices.
It is becoming apparent that we are nearing the limits of what can be achieved with silicon-based technology. To meet global data demands, researchers are now seeking new materials capable of delivering more performance capabilities with less energy required.
Enter spintronics: A quantum leap forward
One such breakthrough is emerging in the field of spintronics (spin electronics). Unlike conventional devices that rely solely on the flow of electrical charge, spintronic technology also harnesses the spin of electrons, a quantum property that gives them magnetic potential.
By encoding binary data using spin states, commonly referred to as “up” or “down”, spintronic devices offer the promise of faster processing speeds, higher data storage density, and significantly lower energy consumption. These advances position spintronics as a leading candidate for powering the next generation of sustainable electronics.
Marco makes magnetic vortexes
Marco Vás, an AINSE PGRA scholar, is contributing to this transformation through his work with ANSTO and the University of Auckland. His research focuses on material optimisation to be better used in developing spintronic devices that are not only faster and more efficient than traditional semiconductors, but also capable of scaling sustainably with digital demand.
While spintronic components are already in use in some modern technologies, Marco is pushing the boundaries further with a novel data storage technique known as skyrmion racetrack memory.
Skyrmions are stable, vortex-like quasiparticles formed by the interaction of atomic magnetic moments under specific conditions of temperature and magnetic fields. These nanoscale structures exhibit remarkable stability, compactness, and energy efficiency, making them ideal for high-density, low-power memory applications.
By leveraging skyrmions, Marco’s research aims to overcome some of the core limitations of traditional storage, such as thermal energy loss and restricted scalability.
The role of skyrmions in advancing future electronics
To bring skyrmion-based racetrack memory closer to practical application, Marco Vás and his collaborators have been working across multiple ANSTO facilities, including the Australian Centre for Neutron Scattering (ACNS) and the Australian Synchrotron, to improve the properties of a promising material: Cu₂OSeO₃ (copper oxide selenite).
Cu₂OSeO₃ is the only known insulating material that can host magnetic skyrmions. Unlike traditional silicon-based materials, its insulating nature reduces energy loss to heat, improving its energy efficiency and thereby reducing its environmental footprint.
However, to make this material suitable for real-world data storage applications, researchers need to understand and control two key factors:
- How its atomic structure influences skyrmion formation, and
- How to modify this structure to optimise the skyrmion formation conditions.
Marco addressed both challenges using a technique called elemental doping, where specific atoms in a material are substituted with atoms of a different element to alter the material’s properties. In this case, larger tellurium atoms were used to partially replace the smaller selenium atoms in Cu₂OSeO₃. This substitution expanded the material’s crystal lattice and weakened the interactions between copper atoms, thereby fine tuning the conditions required for the formation of stable skyrmions.
To confirm the desired structural changes had been achieved, Marco used the Powder Diffraction beamline at ANSTO’s Australian Synchrotron, along with the Wombat and Echidna neutron powder diffractometer beamlines at ACNS. These advanced tools allowed him to accurately characterise the crystal structure of the doped material under varying temperatures and magnetic fields. His results showed that tellurium was successfully incorporated into the crystal structure, slightly increasing the spacing between copper atoms.
To evaluate the impact of this doping on skyrmion behaviour, Marco used the Quokka small-angle neutron scattering (SANS) instrument at ACNS. His experiments revealed that skyrmions still formed in the doped material, at lower temperatures and under weaker magnetic fields than was required in the original undoped material. This result means that using doped Cu₂OSeO₃ lowers the electrical energy requirements to generate the necessary magnetic field for skyrmion formation.
Overall, tellurium doping improved the energy efficiency of skyrmion formation without compromising the material’s essential magnetic properties, demonstrating a significant step forward in developing low-power, high-density memory devices using spintronic technologies.
Innovative materials that will drive a sustainable future
From the digital cloud to the core of our devices, the future of sustainable computing depends on reimagining the materials that make it all possible. Spintronic technologies, powered by electron spin and magnetic interactions, offer a compelling path forward.
Marco’s research contributes to the development of promising new-generation materials that could transform how we store, process, and manage the world’s growing digital footprint.
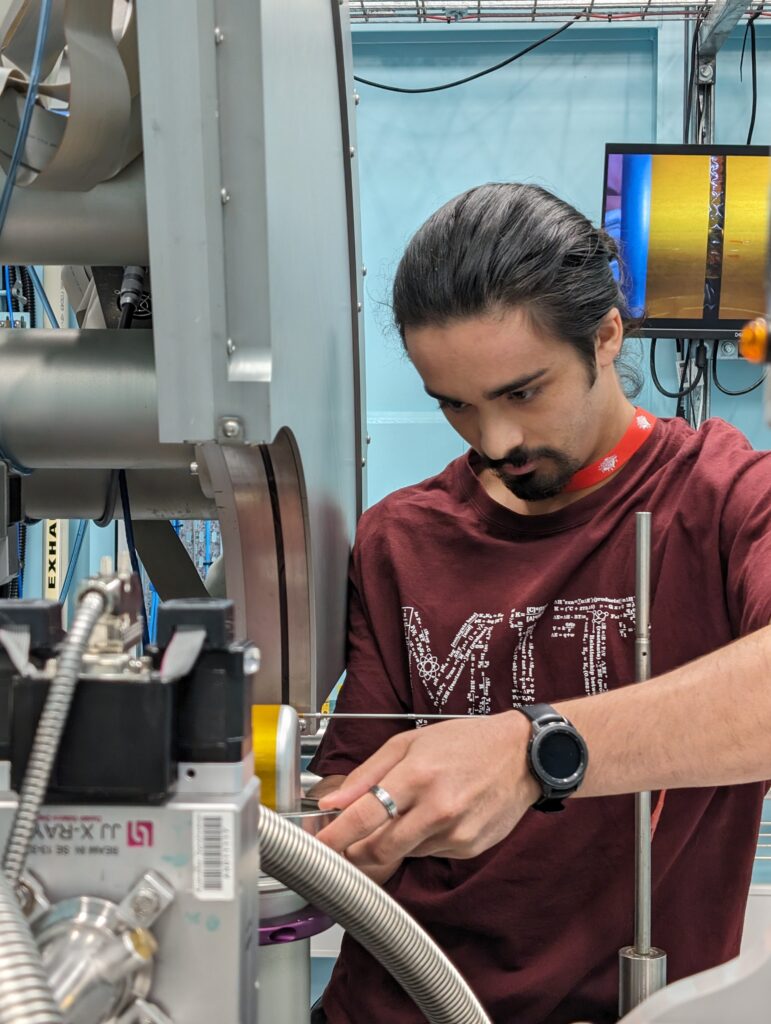
AINSE are proud to spotlight Marco for his innovative work!
If you, like Marco, are interested in tackling major problems with atomic solutions, visit ainse.edu.au/scholarships to see how AINSE can support you.
To keep exploring AINSE’s research spotlights head to ainse.edu.au/research-spotlight.
Stay tuned for our next Magnetic May article, featuring Kyle Portwin’s research on enhancing thermo-electric materials with magnetic nanoparticles.
Follow ainse_ltd on Instagram, Facebook, Threads and LinkedIn to keep up to date with upcoming events and research spotlights.